没有合适的资源?快使用搜索试试~ 我知道了~
Resolution-enhanced intensity diffraction tomography in high num...
0 下载量 36 浏览量
2021-02-22
14:09:58
上传
评论
收藏 4.43MB PDF 举报
温馨提示
We propose label-free and motion-free resolution-enhanced intensity diffraction tomography (reIDT) recovering the 3D complex refractive index distribution of an object. By combining an annular illumination strategy with a high numerical aperture (NA) condenser, we achieve near-diffraction-limited lateral resolution of 346 nm and axial resolution of 1.2 μm over
资源推荐
资源详情
资源评论























Resolution-enhanced intensity diffraction
tomography in high numerical aperture label-free
microscopy
JIAJI LI,
1,2
ALEX MATLOCK,
3
YUNZHE LI,
3
QIAN CHEN,
1
LEI TIAN,
3,4
AND CHAO ZUO
1,2,
*
1
School of Electronic and Optical Engineering, Nanjing University of Science and Technology, Nanjing 210094, China
2
Smart Computational Imaging Laboratory (SCILab), Nanjing University of Science and Technology, Nanjing 210094, China
3
Department of Electrical and Computer Engineering, Boston University, Boston, Massachusetts 02215, USA
4
e-mail: leitian@bu.edu
*Corresponding author: zuochao@njust.edu.cn
Received 31 July 2020; revised 25 September 2020; accepted 30 September 2020; posted 2 October 2020 (Doc. ID 403873);
published 12 November 2020
We propose label-free and motion-free resolution-enhanced intensity diffraction tomography (reIDT) recovering
the 3D complex refractive index distribution of an object. By combining an annular illumi nation strategy with a
high numerical aperture (NA) condenser, we achieve near-diffraction-limited lateral resolution of 346 nm and
axial resolution of 1.2 μm over 130 μm × 130 μm × 8 μm volume. Our annular pattern matches the system’s
maximum NA to reduce the data requirement to 48 intensity frames. The reIDT system is directly built on
a standard commercial microscope with a simple LED array source and condenser lens adds-on, and promises
broad applications for natural biological imaging with minimal hardware modifications. To test the capabilities of
our technique, we present the 3D complex refractive index reconstructions on an absorptive USAF resolution
target and Henrietta Lacks (HeLa) and HT29 human cancer cells. Our work provides an important step in in-
tensity-based diffraction tomography toward high-resolution imaging applications.
© 2020 Chinese Laser Press
https://doi.org/10.1364/PRJ.403873
1. INTRODUCTION
The refractive index (RI) of biological cells and tissues plays a
crucial role in biomedical imaging as it captures the physiologi-
cal characteristics and morphological features of a sample [1–3].
Imaging 3D biological samples with high resolution, however,
remains a challenging task. Fluorescence-based 3D imaging
methods, such as confocal microscopy [4] and multiphoton
microscopy [5], already provide excellent optical sectioning
and enable super-resolution 3D volume imaging of nanoscale
subcellular structures. Unfortunately, the exogenous labels
(e.g., dyes and fluorophores) in these techniques can be photo-
toxic and cause photobleaching due to the absorption of the
excitation light [6] and can artificially alter the sample’s behav-
ior and cellular structure [7]. Here, we introduce a resolution-
enhanced intensity diffraction tomographic (reIDT) technique
based on a conventional bright-field microscope and a high-
density LED array illumination unit with sparse annular illu-
mination. The imaging method is label-free, motion-free, fast,
and enables the high-resolution quantitative recovery of 3D in-
trinsic structural features at the single cell level.
Quantitative phase imaging (QPI) [3] is an often-used tech-
nique to recover biological cells with high contrast in their
natural states. QPI reconstructs a quantitative image of the
sample-induced two-dimensional (2D) optical path thicknesses
or phase delay based on interferometry [8–11] and noninter-
ferometry [12–18] methods. However, these phase maps evalu-
ate the sample’s total optical delay along the integrated direction
over a thin layer and provide limited volumetric information
about the object’s internal structure. To capture this informa-
tion and evaluate thicker specimens, tomographic methods
recovering 3D information are required. Recently developed
3D RI tomography techniques enable the reconstruction of
the sample’s 3D RI distribution to visualize intracellular struc-
tures, and the related 3D imaging techniques can be largely
categorized into two classes, interferometry-based and inten-
sity-only methods.
Optical diffraction tomography (ODT) is the most widely
used interferometry-based 3D QPI technique [19,20].
Standard implementations of ODT use either a rotating sample
[21] or a scanning laser beam [22–24] to capture the angle-
specific scattering arising from the sample. A 2D complex scat-
tered field containing the object’s phase and amplitude infor-
mation is directly recorded as digital interferograms under
various illumination angles, and a tomographic reconstruction
algorithm is applied to recover the sample’s 3D RI distribution.
The interferograms are captured using either an off-axis
1818
Vol. 8, No. 12 / December 2020 / Photonics Research
Research Article
2327-9125/20/121818-09 Journal © 2020 Chinese Laser Press

[20–24]orcommonpath[25–27] holographic microscope, and
numerous ODT approaches [22–24] have been applied in bio-
medical imaging for achieving quantitative, high-resolution 3D
imaging. Hence, ODT can avoid the complex sample-prepara-
tion protocol and photobleaching effects of fluorescence while
still recovering a sample’s 3D morphology, and this label-free
3D reconstruction method has found great success in visualizing
individual unlabeled cells with quantitative refractive indices.
For intensity-only techniques, existing IDT techniques in-
volve taking multiple defocused intensity images under sym-
metric [28–31] or asymmetric [32,33] illuminations, and the
3D RI map is recovered using deconvolution [34]. Techniques
such as differential interference contrast acquire through-focus
intensity stacks to extract the 2D phase information at multiple
focus planes, and the 3D RI distribution is reconstructed using
gradient phase information [35]. Recent works further incor-
porate modulated partially coherent sources (e.g., annular shape
and Gaussian shape) to enhance the response of the 3D transfer
function for noise-robust 3D tomographic results [30,31].
However, these methods often require sample or objective
mechanical scanning along the axial direction to capture a
through-focus intensity stack. This scanning limits the setup’s
acquisition speed and requires large datasets with hundreds of
intensity frames for object recovery. Alternatively, IDT ap-
proaches have been developed allowing 3D RI information re-
covery using angle d illumination-only intensity measurements
without any mechanical scanning [36–42]. These methods ap-
ply single-scattering approximation-based models enabling
direct 3D object reconstruction with a closed-form solution
[39–41]. Iterative algorithms based on multiple scattering
models using the multislice beam propagation method have
also been developed to recover strongly scattering samples
[37,43,44]. One drawback of most of these existing techniques
is the large number of required intensity measurements that
consist of hundreds of intensity images per volume. The iter-
ative reconstruction algorithm further prolongs the whole 3D
imaging process and can become computationally expensive.
Here, we present a reIDT setup that utilizes a high-density
LED array hardware and a high numerical aperture condenser
to significantly improve the achievable resolution of the single-
scattering-based IDT technique using a larger objective
(NA
obj
0.9). Compared to existing single-scattering-based
IDT techniques [39–41 ], we improve the imaging resolution
to near-diffraction-limited lateral resolution of 346 nm.
Specifically, the achievable axial resolution is extended from
3.4 μm to 1.2 μm enabling single-cell-level 3D structure im-
aging. By introducing a condenser lens to the setup, reIDT im-
proves the illumination efficiency and reduces the system’s
required exposure time. The annular illumination pattern op-
timally fitted with the circular microscope pupil also reduces
the image requirement to improve the system’s speed. Finally,
we show that the present technique provides high-resolution,
volumetric, subcellular information and morphology analysis
on multiple biological samples. We recover macroscale cellular
membrane structures, subcellular organelles, and micro-
organism tissues to demonstrate that our technique has poten-
tial as a fast and high-resolution 3D imaging tool for biological
samples.
2. THEORY AND METHOD
A. Principle of reIDT
As depicted in Fig. 1(a), the proposed reIDT imaging system
contains a high-density LED array illumination unit and an
Abbe condenser attached to a commercial bright-field micro-
scope. By placing the LED array in the front focal plane of
the condenser lens, each LED source located at the condenser
aperture diaphragm provides spatially partially coherent illumi-
nation in the form of a plane wave at a unique illumination
angle on the sample. The annular pattern is displayed on the
LED array, and ring-shape illumination (radius R, illumination
numerical aperture NA
illum
) is matched with the objective lens’s
pupil aperture NA
obj
properly (i.e., NA
illum
≈ NA
obj
), as illus-
trated in Fig. 1(b). The specimen is placed in the objective’s
focal plane and illuminated by each LED in the ring array.
The intensity from each illumination is recorded on a camera
which captures the interference of the object scattered field and
the unperturbed illuminating field under the single-scattering
approximation [Fig. 1(c)].
By quantifying the Fourier space information under the first
Born approximation, the phase and absorption transfer func-
tions (TFs) of the IDT linear model can be derived [39], and
the object’s complex permittivity distribution can be linearly
related to the measured intensity spectrum. The 4D TFs in
Fig. 1(d) contain slice-wise (2D) phase and absorption transfer
functions at different depths for each illumination angle, and
the exact form of the TFs can be found in Eq. (5) and Eq. (6).
Importantly, since the TFs are confined to each slice, the scat-
tering information from different sample slices is decoupled.
This decoupling allows us to use a computationally efficient,
slice-based deconvolution procedure to reconstruct the cross-
sectional RI distribution one slice at a time. The raw intensity
image set is captured by varying the illumination angles, and
each intensity spectrum of the obliquely illuminated images ex-
hibits a pair of circular Fourier regions. The pupil function fil-
ters the incoming scattered field to this circular shape, while the
oblique illumination’s transverse frequency and its conjugate
translate the scattered field in opposite directions in the fre-
quency domain [Fig. 1(e)]. The lateral cutoff frequency
envelope is indicated by the white dashed circle with the
LED source’s illumination angle (k
cutoff
∼2NA
obj
∕λ)in
Fig. 1(e). By combining the LED position self-calibration al-
gorithm, the accurate transverse frequency of incident illumi-
nation can be obtained and used for modeling the 4D TFs.
Finally, a fast and efficient slice-wise 3D deconvolution algo-
rithm with a closed-form solution [Eqs. (7) and (8)] is imple-
mented to recover the sample’s complex 3D RI distribution.
Since the IDT model is only valid for bright-field measure-
ments, the system still suffers from the missing cone problem
in the axial direction, resulting in the axial elongation present in
the reconstruction. Figure 1(f) illustrates the frequency support
region of the proposed reIDT method in k-space.
In practice, using a high-NA objective and illumination
scheme cannot assume paraxial imaging conditions. As pro-
posed by Sheppard and Gu et al. [45–47], high-angle micro-
scope system s usually incorporate an apodization function
introduced by the system satisfying the sine condition. Thus,
the investigation of the objective aperture function Pu
Research Article
Vol. 8, No. 12 / December 2020 / Photonics Research 1819
剩余8页未读,继续阅读
资源评论

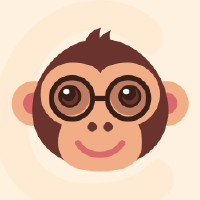
weixin_38672794
- 粉丝: 5
- 资源: 924
上传资源 快速赚钱
我的内容管理 展开
我的资源 快来上传第一个资源
我的收益
登录查看自己的收益我的积分 登录查看自己的积分
我的C币 登录后查看C币余额
我的收藏
我的下载
下载帮助


最新资源
- comtrade录波文件格式详解-1999中文版
- inode for mac客户端,H3C
- Python 实现CSO-BP布谷鸟优化算法优化BP神经网络多输入单输出回归预测的详细项目实例(含完整的程序,GUI设计和代码详解)
- PFC-FLAC耦合断层模型简化版:球体与有限元层间交互的干货指南,PFC-FLAC耦合模型简化版:带有断层特性的有限元分析与实践教程,该模型是“PFC- FLAC耦合带有断层的模型”的简化版: 即p
- 2000-2022年上市公司人工智能水平数据/上市公司人工智能词频统计数据(年报词频统计).xlsx
- 基于DSP TMS320F28335的Matlab Simulink嵌入式模型:自动生成CCS工程代码实现永磁同步电机双闭环控制,基于Matlab Simulink开发的TMS320F28335芯片嵌
- Python 基于扩散因子搜索的GRNN广义回归神经网络时间序列预测的详细项目实例(含完整的程序,GUI设计和代码详解)
- Python 实现SA-ELM模拟退火算法优化极限学习机时间序列预测的详细项目实例(含完整的程序,GUI设计和代码详解)
- COMSOL模拟中考虑浆液粘度时变性的随机裂隙注浆过程:多孔介质与优势裂隙通道内的流变行为研究,COMSOL模拟浆液在多孔介质与裂隙中复杂流动行为的时变粘度特性研究,COMSOL注浆( 1coms
- Python 实现ELM极限学习机时间序列预测的详细项目实例(含完整的程序,GUI设计和代码详解)
- numpy-2.2.0-cp311-cp311-win32.whl
- VPet,虚拟宠物收集的资料
- 基于Comsol仿真模型的锂枝晶生长过程研究:多场耦合与C++程序模拟的元胞自动机法及LBM对流影响分析,基于Comsol仿真模型的锂枝晶生长过程研究:多场耦合与C++程序模拟的元胞自动机法及LBM对
- TMS320F28P550SJ9学习笔记5:结构体寄存器方式配置 LED
- MATLAB驱动直线电机创新应用:仿真示波器曲线与数据分析验证法效能,MATLAB直线电机仿真与数据验证:创新方法的有效证明及文档化展示,MATLAB直线电机创新点,通过仿真示波器的曲线或者数据能证
- linux与unix shell编程指南
资源上传下载、课程学习等过程中有任何疑问或建议,欢迎提出宝贵意见哦~我们会及时处理!
点击此处反馈



安全验证
文档复制为VIP权益,开通VIP直接复制
